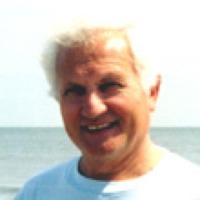
Robert Malcuit
Although I retired from teaching in 1999, I am still pursuing some research interests in the earth and planetary sciences. Currently my research pursuits are: (1) gravitational capture potential for planets, (2) explanation of many of the major features of the Earth and Moon via the process of gravitational capture of a lunar-mass planetoid from a heliocentric orbit into a geocentric orbit in a prograde orientation, (3) explanation of the major features of planet Venus via a gravitational capture scenario in which a 0.5 moon-mass body is captured from a heliocentric orbit into a venocentric orbit in the retrograde orientation, (4) explanation of many of the features of the Neptune-Triton system via gravitational capture of a triton-mass body in the retrograde orientation, (5) explanation of several of the unique features of the Earth in terms of a capture origin of the Earth-Moon system and the subsequent evolution of the lunar orbit to the present condition.
Research
Gravitational Capture Potential for Planets
In the era of 1990-1993, two physics/computer science students (Wentoa Chen, DU,’92 and Albert Liau, DU,’93) made minor corrections to the numerical code and developed programs for plotting tidal amplitudes as well as tidal ranges directly from the geocentric orbital data. In general, these three very talented undergraduate students (Mehringer, Chen, and Liau) were super important in the development of this planetoid capture project. It is interesting to note here that of all the articles in the planetary science literature on gravitational capture (or articles mentioning the process before 1987) no investigator had done a successful numerical simulation of capture. I think the main reason that it had not been done is because of some fundamental paradoxes that are associated with gravitational capture processes in general.
(NOTE: A good summary of lunar origin models can be found in a book by Peter Cadogan published in 1981 titled “The Moon: Our Sister Planet”. This book was published before the Giant Impact Model became dominant.)
The first paradox is that the planetoid (the smaller body) must absorb most of the energy for its own capture. Successful capture is a matter of h’s and Q’s. The h (displacement Love number) is a measure of the deformability of the planet or planetoid and the Q (specific dissipation factor) is a measure of the quantity of the energy stored by tidal distortion during a close gravitational encounter that is subsequently dissipated during a single encounter (a tidal oscillation). The fraction of stored energy that is dissipated during one tidal oscillation is 1/Q. A reasonably warm lunar-mass planetoid could have an h in the range of 0.1 to 0.3. For capture the Q of this planetoid must be very low: 1, 2, or 3. In contrast to the planetoid, the planet is a passive bystander furnishing a strong gravitational field for tidal deformation of the planetoid. The h of the Earth at present is about 0.54 (a very deformable body). The Q of the Earth is very high (about 200). The h and Q for the primitive Earth (about 4.0 billion years ago) would not have been greatly different (perhaps h=0.7 and Q=200). The bottom line is that the earth, at no time in its history, would be an effective energy sink for tidal capture.
A second paradox is that larger planetoids are more capturable than smaller ones. The reason for this is that as the planetoid radius decreases, the h for storing the energy for capture increases. Thus, if all candidate planetoids of lunar density have a low Q value, only those larger than about 0.2 lunar mass can temporarily store, by tidal distortion, the energy for their own capture.
A third paradox is that "cool" planetoids are more capturable than “hot” planetoids. Martin Ross and Gerald Schubert did a set of calculations at UCLA in the middle 1980’s on “Q vs. Viscosity” of a planetoid. Their conclusions were that a low Q is associated with an intermediate value of planetary viscosity. In other words, if a body is too cold, it will be too rigid (high viscosity) to absorb much energy. If a body is too hot (low viscosity), the body can be easily deformed but it will be too “mushy” to dissipate more than a small fraction of the tidal energy of any one tidal oscillation. The low Q values, then, are associated with an intermediate viscosity value. A reasonable assumption is that for a lunar-like planetoid the intermediate viscosity condition would be when the lowest melting component of the lunar magma ocean (probably at a depth of about 200 km) would be just below the melting point. Thus, with an h in the range of 0.20 to 0.25 and a Q near 1, sufficient energy could be dissipated for capture during one tidal oscillation. These are the planetiod body conditions necessary for tidal capture.
I have now calculated and plotted literally thousands of successful capture scenarios and about as many encounter sequences that result in collision on some subsequent encounter as well as a number of escape sequences. The results of this large quantity of capture, collision and escape scenarios have led to the concept of a stable capture zone (SCZ). A stable capture zone is a restricted region of parameter space (planet anomaly vs. planetoid orbital eccentricity) in which capture can occur IF sufficient energy is dissipated in a combination of interacting bodies to insert the body of the planetoid into a geocentric orbit. The philosophical value of the geometry of these SCZs is that one can estimate (calculate) directly the probability of capture when the h and Q values are within the permissible range for gravitational capture.
I have found that there are four stable capture zones for each planet-planetoid combination: two prograde SCZs and two retrograde SCZs. That is, one can capture a planetoid from an orbit that is slightly larger or slightly smaller than the orbit of the planet and the encounter can be in a counterclockwise direction (prograde) or in a clockwise direction (retrograde) as viewed from the north pole of the Solar System.
Other Problems to Be Addressed
Two other problems that need to be addressed by a Gravitational Capture Model for the Origin of the Earth-Moon System are (1) the place of origin of the pre-capture planetoid (Luna) and (2) the similarity of oxygen isotope ratios of the Earth and Moon. Without going into technical details and to shorten the story considerably, a favorite place of origin at present is inside the orbit of planet Mercury at about 0.1-0.2 AU (astronomical unit). Such a place or origin is compatible with the anhydrous and refractory nature of lunar rocks (no water of hydration). And according to Evans and Tabachnik in a 1999 article in the journal Nature, planetoid orbits can be stable in this region of space for hundreds of millions of years. I think, then that this region is a good place for the formation of refractory planetoids.
The Similarities of Oxygen Isotope Ratios between Earth and Moon:
The problem of the similarity of oxygen isotopes ratios between Earth and Moon is a bit more challenging. It is known that Earth, Moon, enstatite chondrites, and the HED asteroids all have oxygen isotope ratio similarities. Mars and a few other asteroids that we think we have samples from via meteorites, are enriched in the heavier oxygen isotopes. However, there is no known information from Venus or Mercury that would relate to whole body oxygen isotope ratios, and until there is, we will not know what constitutes a tread in the inner solar system. My prediction is that the oxygen isotope ratios for planets Mercury and Venus will be very similar to those of planet Earth and Luna (the Moon).
(NOTE: A good summary and discussion of the oxygen isotope ratios of various solar system bodies as well as information on many other lunar and planetary science topics is found in a book by Ross Taylor published in 2001.)
Perhaps a more consistent index for deciphering a place of origin of Luna is what I call the Potassium Index for inner Solar System bodies. Humayun and Clayton published a paper in 1995 in which they had a plot of Potassium content of a body divided by the Uranium content of the body. This K-index scale was on the vertical axis and the positions of several planetary bodies were plotted on the rectangular plot. The body with the lowest value on the plot was the Angrites meteorites, then Luna, then the HED group of meteorites (from Vesta), then Earth, then Mars, then Ordinary Chondritic meteorites, and at the top of the chart were the Carbonaceous Chondritic meteorites. The authors of the article suggested that this plot might relate to the temperature of formation of the solar nebular material (minerals) that condensed from the solar nebula to form the various bodies. For a more extensive discussion of both oxygen isotope ratios and the K/U index, I refer you to a book by Ross Taylor published in 2001 on Solar System Evolution.
The X-Wind Model and the Place of Origin of Luna:
Frank Shu (emeritus professor at UC-Berkeley and San Diego) has been working on models for the early history of sun-like stars (for example, Lada and Shu, 1990; Shu et al., 1991, Shu et al, 2000, and Shu et al., 2001). The name of the model is derived form the geometry of magnetic flux lines that intersect in the form of the letter “X”. They refer to their model as the X-Wind Model. This X-Wind Model appears to explain very successfully the origin of calcium-aluminum inclusions (CAI’s) in meteorites but has been less successful in explaining the origin of chrondrules. This X-Wind would operate during the early history of the Sun before it settles into the main sequence of burning. There are some high-temperature pulses involved which result from reconnection of solar magnetic field lines and Shu thinks that there is enough energy associated with this flaring action to evaporate Oxygen-16 rich dust near the inner edge of the solar accretion disk and thus enrich this region in Oxygen-16 (light oxygen). I think that this excess O-16 could enrich the preplanetary nebular cloud and subsequent planetoid-building materials, all the way out to the vicinity of planet Earth. As mentioned earlier, a prediction based on the X-Wind Model is that Venus and Mercury would fall along the same oxygen-isotope trend as the Earth and Moon. Thus, there is a possibility that the Gravitational Capture Model may be compatible with the similarity of oxygen-isotope ratio patterns of Earth and Moon.
Planet Venus
Planet Venus has about the same radius as Earth and it is just a bit closer to the Sun but it has radically different features. The rotation rate is very slow in the retrograde (clockwise) direction, there is virtually no obliquity (tilt angle), and it has a very dense atmosphere composed mainly of carbon dioxide. It also has no old rocks on its surface, nothing older than 1.0 billion years and maybe only one half that age. An obvious question is: why the radical differences between sister planets?
A retrograde capture scenario for planet Venus may help explain the differences. The scenario goes something like this. Planet Venus captures one of these refractory planetoids (a planetoid that I have named Adonis) formed in this belt between Mercury and the Sun into a retrograde venocentric orbit. The highly elliptical orbit then circularizes in a few tens of millions of years after capture (mainly due to energy dissipation in the planetoid) and continues to become smaller after circularization (mainly because of rock and ocean tidal energy dissipation is mainly in the planet). The time scale of interaction is long (billions of years), but the trend is for the circular orbit to get smaller and smaller as the prograde spin rate of the planet decreases and the tides (both ocean and rock tides) become higher in amplitude and frequency. Eventually, after about 3.0 to 3.5 billion years of orbital evolution, the satellite is in a small circular orbit and stirs the mantle so much, in a unidirectional manner, that the original crust is subducted into the mantle and the planet gets a global resurfacing of basalt. The satellite eventually comes so close to the planet that it is at the Roche limit for a solid body. The satellite breaks up in orbit (within about 1.6 venus radii) and the pieces fall to the surface of Venus via atmospheric drag. The dense atmosphere would consist mainly of carbon dioxide, the main volcanic gas generated by the super (planet-wide) volcanic action. A satellite with about one-half the mass of Earth’s Moon would be sufficient to despin Venus from a primordial rotation rate of about 15 hours/day prograde to zero and then cause it to rotate very slowly in the opposite (retrograde) direction.
If this story holds up for planet Venus, then Earth and Venus have something in common: they both have gravitationally captured satellites. The difference is in the details. Earth captured a planetoid in the prograde direction and Venus captured a planetoid in the retrograde direction. The results for the evolution of the "sister planets" are radically different!
(NOTE: A retrograde capture scenario was first proposed by Fred Singer in a 3-page article in the journal Science in 1970. But I am apparently the only one to pursue the geological consequences of such a retrograde capture scenario.)
For more information on the origin of the Earth’s Moon and the Origin of the Earth-Moon system I refer you to my 2015 book:
- Malcuit, Robert J., 2015, The Twin Sister Planets, Venus and Earth: Why are they so different?: Springer International Publishers, 401 p.
(Note: You can get much information about the contents of the book by googling “Malcuit, Twin Sister Planets, Springer”.
Planet Neptune
Planet Neptune is the most distant icy-gassy planet in the Solar System. It has two sizeable satellites that have been known for many years and many smaller ones that have been discovered recently via the Ulysses mission to he outer planets. Nereid is a small satellite in a large, somewhat eccentric prograde orbit and does not pose much of a problem for explanation. Triton, in contrast, is a large satellite in a small, circular retrograde orbit that is inclined about 20 degrees to the plane of the planets. Triton is a major problem to be explained!
Most investigators agree that Triton was somehow captured into a retrograde orbit. The currently most popular explanation is that published by Peter Goldreich and others in 1989 in the journal Science suggesting that Triton is a product of collisional capture. In this model, planetoid Triton, would just happen to crash into a small existing satellite as it approaches planet Neptune. The orientation of the existing satellite and that of planetoid Triton, in this model, was just right to take enough energy from planetoid Triton’s orbit to change it from a heliocentric orbit to an elliptical retrograde neptocentric orbit. Then over geologic time the orbit would circularize via tidal dissipation within the body of Triton. The authors’ suggested place of origin of the planetoid is the Kuiper Belt of icy planetoids, which is located just beyond the orbit of Pluto.
My model for the origin of the Neptune-Triton system has much in common with the above model. The difference is in the capture mechanism. It so happens that icy, triton-mass planetoids can have a low Q value at intermediate viscosity conditions just like rocky ones. Numerical simulations of gravitational capture show that it is possible to capture planetoid Triton into a large, but stable, retrograde orbit by way of energy dissipation within the body of Triton during one close encounter with planet Neptune using very reasonable h and Q values for Triton. The SCZs for retrograde capture are fairly large for encounters from either an orbit slightly larger or slightly smaller than Neptune’s orbit. The SCZs for prograde capture, however, are very limited and much more energy dissipation is necessary for prograde capture. Thus, retrograde gravitational capture is highly favored over prograde gravitational capture for a Triton-mass planetoid. After successful retrograde capture, in this model, the highly elliptical orbit then circularizes over a long period of time (billions of years) with nearly 100% of the tidal energy for orbit circularization dissipated in the body of Triton. Rocky planets are poor absorbers of tidal energy because of a high Q value, but icy-gassy planets are even poorer absorbers because their Q values are an order of magnitude higher than those of their rocky relatives. Thus, the orbit of Triton is essentially “frozen” at its present location and the body of Triton may not coalesce with Neptune in the history of the Solar System.
Chapter 6 of my most recent book (Geoforming Mars) has a well-illustrated section on the details of the retrograde capture of Triton by planet Neptune. The book is:
- Malcuit, Robert J., 2020, Geoforming Mars: How could nature have made Mars more like Earth?: Springer International Publishers, 400+ p.
Chapter 6 also has a section on a model that may explain the origin of Pluto as an escaped satellite of Neptune. This model (scientific story) was proposed by Raymond Lyttleton (Astronomy Professor, Cambridge University, U. K.) in 1936, just 6 years after the discovery of Pluto. My Pluto story is similar to that of Lyttleton, with a few different conditions, but the general theme is the same: Pluto is an escaped satellite of Neptune. In Lyttleton’s model two prograde satellites of Neptune had a gravitational close encounter. One of the satellites was deflected into a retrograde orbit to become Triton and the other satellite was gravitationally ejected from the Neptune system into a heliocentric orbit to become planet Pluto. In my model Triton is gravitationally captured from a heliocentric orbit into a Neptune-centered orbit with significant eccentricity. While undergoing orbit circularization the body of Triton was gravitationally interacting with one of the original satellites of Neptune which was in a prograde orbit. Eventually Triton had a very close, slightly grazing, gravitational encounter with the prograde satellite of Neptune. During the encounter, the retrograde orbit of Triton was altered but remained retrograde and the prograde satellite of Neptune was gravitationally ejected in to an orbit like that of Pluto today. During the encounter, Proto-Pluto, the prograde satellite of Neptune, lost some mass due to the grazing encounter and this mass went into retrograde orbit about Pluto and the Pluto-Charon system was born. My colleagues and I have done limited numerical simulations of the Pluto-Charon story and will be doing more in the near future.
This model for the origin of Pluto as an escaped satellite of Neptune also explains the origin of named small satellites of Pluto, the synchronous spin-orbit coupling of Pluto and Charon, and the ellipticity and inclination or the heliocentric orbit of Pluto relative to other outer Solar System planets. As an added bonus of the model, we think that the prominent feature of Pluto, Sputnik Planitia, is the site of gravitational extraction of the mass of Charon, and the other satellites of Pluto, from Proto-Pluto.
(Historical note: Lyttleton published his model in 1936, the year I was born. Very seldom does one get to add onto or extend or enhance a model that was proposed in his or her birthyear!)
Also included in Chapter 6 of the “Geoforming Mars” book is a section on the long-standing solar system mystery of the high tilt angle (98 degrees) of planet Uranus and its extensive satellite system. A recent explanation by Slattery et al. (1992) is that it was the result of a collision of a 1 to 3 Earth-mass body with Uranus. However, two Japanese scientists (Kubo-Oka and Nakazawa, 1995) suggested a much less catastrophic model. They proposed that capture of a 20 moon-mass object (about 0.2 mars-mass and about 0.02 Earth mass) by Uranus in a retrograde direction could also result in such a tilt angle but they could not demonstrate that retrograde capture of such an object was physically possible. Since we have a numerical simulation program for capturing bodies from heliocentric into planetocentric orbit, my colleagues and I are in the process of putting a “front-end” on their model. We are now in the process of defining the stable capture zones for a Uranus-mass planet and a 20 moon-mass planetoid that I have named Discordia. The general scheme of planetoid capture, post-capture orbit evolution, circular orbit evolution, and progressive increase of tilt angle as the retrograde circular orbit decreases in radius are outlined in a section of Chapter 6 of the “Geoforming Mars” book.
Back to Planet Earth
It appears difficult for planetary scientists (including geologists, geophysicists, astronomers, etc) to think of planet Earth as part of the Earth-Moon system. But the Moon seems to have had a subtle, but emphatic, effect on the history of our planet. For example, the rotation rate on a moonless Earth would be in the range of 12 to 14 hours per day. The tidal mechanism, mainly the ocean tides operating in the past 600 million years, has slowed the rotation to the present, very comfortable, 24 hours/day. A number of earth and planetary scientists have also suggested that unidirectional mantle rock convection currents may cause some unidirectional trends in the movement of tectonic plates. They suggest that these plate movements may be powered by the unidirectional operation of earth (rock) tides (which really have been operating ever since the Moon got associated with planet Earth). An article by Nelson and Temple in the 1972 volume of the Bulletin of the American Association of Petroleum Geologists is interesting reading. A recent (2000) book by Robert Bostrom (University of Washington) titled Tectonic Consequences of Earths Rotation is also very interesting reading. A recent summary of the possible influence of earth tides on plate tectonics was published by Scoppola and others in 2006 in the Geological Society of America bulletin. Plate movements over geologic time have had a very significant effect on biological evolution. Some of these biological consequences caused by the evolution of the Earth-Moon system are covered in a book by Peter Ward (geologist) and Donald Brownlee (astronomer) from the University of Washington. The title is Rare Earth: Why Complex Life is Uncommon in the Universe published in 2000. In general, I think that the study of the subtle rock and ocean tidal effects of the Moon on the Earth is a potentially fruitful endeavor!
Planet Orbit - Lunar Orbit Resonances
Still another interest of mine along the “rare earth” theme is on planet orbit – lunar orbit resonances and their possible geological effects on our planet. In recent years I have been working on the long-term tidal evolution of the Earth-Moon system from the time of capture (about 3.9 billion years ago) to the present. I am searching for eras in the history of the system in which the lunar orbit may enter into resonances with the orbital motion of planets, mainly Jupiter and Venus. The main concept here is that, for a resonant condition, the period of the perigean (or apsidal) cycle of the satellite (the prograde progression of the position of the moon at perigee) is equal to, or nearly equal to, the period of the heliocentric orbit of the perturbing planet. A four-body (sun, earth, moon, jupiter) numerical program is used for calculation of a Jupiter Orbit—Lunar Orbit resonance. Such an orbital resonance causes a forced eccentricity of the lunar orbit consequently causing an era of higher than normal ocean and rock tides on both the planet and satellite. For the case of Jupiter this orbital resonance would be most effective when the Moon is at an orbital distance of about 53.4 earth radii (probably between 1.0 and 0.6 billion years before present). This is a time of significant events in the rock record of Earth:
(1) There is good evidence of at least two major “global” glaciations.
(2) There is the development of several major continental rift zones.
(3) There is a major change in the pathway of organic evolution from an algal-bacterial regime to a world with metazoans.
(4) There is an abundance of tidal rhythmite sequences in rock units of this era.
(NOTE: The first mention of a Jupiter Orbit – Lunar Orbit resonance was in an article by Peale and Cassen in the journal Icarus in 1978. They suggested, and I am paraphrasing, that if the resonance is stable, there could be significant thermal implications for both Earth and Moon. I am the only one to my knowledge to pursue these geological and lunar ramifications.)
I am also exploring the possible effects of Venus Orbit – Lunar Orbit resonances. These planet orbit—lunar orbit resonances would be somewhat weaker gravitational influences than the Jupiter-powered resonance. If any of these Venus-induced orbit resonances are stable enough over a significant period of time, they could cause a significant increase in eccentricity of the lunar orbit and thus could cause increased rock and ocean tidal activity in the equatorial zone of the planet. The enhanced tidal action, in turn, could cause some favorable environmental conditions for evolution of life forms on the planet during certain eras of our planet’s history. During these eras of increased orbital eccentricity, the global tectonic regime would also be affected by an increase in “tidal vorticity induction”, a process described in Robert Bostrom’s book mentioned above.
Chapter 8 of the “Twin Sisters” book (2015) has a well-illustrated explanation of the concept of planet orbit – lunar orbit resonances and their probable imprint on the geological records of both Earth and Moon.
The Cool Early Earth Model
Finally, there is another model in which I have a special interest – the cool early earth model. John Valley (Univ. of Wisconsin) and coworkers suggest that the near constant range of oxygen-isotope ratios in zircon crystals implies somewhat temperate condition on Earth from about 4.4-2.6 billion years ago). This time-frame includes most of the Hadean Eon as well as all of the Archean Eon. In the article (Valley et al., 2002) the authors question whether the Giant-Impact Model for the origin of the Earth’s moon is compatible with this new information because the putative earth-shattering impact which results in the formation of the Moon must happen and the Earth must cool to a condition where oceans can form (a temperature of about 200 degrees C) before 4.45 billion years ago. Valley et al. (2002) suggest that maybe a planetoid capture model should be considered for the origin of the Earth-Moon system.
(NOTE: In addition to the Valley et al. (2002) article, there is a very well illustrated article by John Valley in the Oct. 2005 issue of Scientific American and a good summary of the zircon crystal information in the journal Elements in 2006).
My gravitational capture model which features capture at about 3.9 billion years ago is very compatible with the cool early earth model. In this capture model the Earth would be moonless from the time of its origin until the time of capture. Thus, the planet could accrete, the metallic core could settle to the center of the planet, the planet could cool, and ocean water could gradually accumulate on the surface as the atmospheric temperature decreases. Another factor that could aid in this cooling is that solar radiant energy would be about 20% less during this era than at present (Valley, 2006). The capture episode would be registered as a high temperature event on the captured planetoid because most of the energy for capture must be dissipated, over a short period of time, in that body. In great contrast, the Earth is a passive bystander in this model. Indeed, the rock and ocean tides on Earth are high and irregular for a geologically brief era, and much of the primitive crust would be recycled into the mantle in the equatorial zone of the planet. But the temperature of the earth’s interior is increased very little by the capture episode and the crust in the polar regions is sheltered from the tidal deformation (which occurs mainly in the equatorial zone of the planet). In other words, surface conditions on Earth are affected significantly, but the thermal regime of the surviving crust (and the associated zircon crystals) as well as the ocean-atmosphere system are not greatly affected.
I am currently working on a model for the petrologic evolution of the primitive crust of the Earth during this “cool early earth” time in Earth History (about 4.567 Ga to the time of Lunar Capture at about 3.95 Ga). The general pattern of this petrologic model is along the line of Bedard (2006) and it is consistent with the Hadean-age zircon crystals which are the only currently identified mineral survivors of this Hadean-age crust of planet Earth.
Planet Mars
My most recent book project is best described as an exercise in “theoretical planetology” (Geoforming Mars: How could nature have made planet Mars more like Earth?). The general idea is that Mars has nearly all of the chemical and physical entities of the Earth but on a smaller scale. So why is Mars so “dusty” and “cold” and Earth so “pleasant”?
In the book I discuss five basic scenarios of planet evolution for Mars with four of the five scenarios involving large satellites relative to the mass of Mars. The Mars-satellite mass ratio is about that of the Earth-Moon system in these theoretical models.
The first scenario is for the Mars that we have today: no recognizable life or fossil forms that we know of but many of the features of planet Earth, simply on a smaller scale, and NO sizeable satellite. The next four scenarios involve large satellites: two featuring prograde capture scenarios and subsequent evolution of the planet-satellite system and two featuring retrograde capture scenarios and subsequent evolution of the planet-satellite system. All four of these planet-satellite scenarios are favorable for organic evolution on a mars-mass planet for at least a segment of the lifetime of the planet.
The general theme of the book is that if we are searching for habitable exoplanets, we should be looking for terrestrial planets with somewhat massive satellites relative to the size of the planet and, of course, liquid water on the surface for some portion of the cycle of the seasons on the planet.
References Cited
Five publications that relate to gravitational capture issues:
- Malcuit, R. J., Winters, R. R., and Mickelson, M. E.,1977, Is the Moon a captured body?: Abstracts Volume, Eighth Lunar Science Conference, p. 608-610.
- Winters, R. R., and Malcuit, R. J., 1977, The Lunar Capture Hypothesis Revisited: The Moon, v. 17, p. 353-358.
- Malcuit, R. J., Mehringer, D. M., and Winters, R. R., 1989, Numerical simulation of gravitational capture of a lunar-like body by Earth: Proceedings, 19th Lunar and Planetary Science Conference, Cambridge Univ. Press and Lunar and Planetary Institute (Houston), p. 581-591.
- Malcuit, R. J., Mehringer, D. M., and Winters, R. R., 1992, A gravitational capture origin for the Earth-Moon system: Implications for the early history of Earth and Moon: in Glover, J. E., and Ho, S. E., eds, The Archaean: Terrains, Processes and Metallogeny: Gelogy Dept. (Key Center) and Univ. Extension, The Univ. of Western Australia, Publication No. 22, p. 223-235.
- Malcuit, R. J., and Winters, R. R., 1996, Geometry of stable capture zones for planet Earth and implications for estimating the probability of stable gravitational capture of planetoids from heliocentric orbit: Abstracts Volume, 27th Lunar and Planetary Science Conference, Lunar and Planetary Institute (Houston), p. 799-800.
One publication on the retrograde capture of a satellite for Venus:
- Malcuit, R. J., and Winters, R. R., 1995, Numerical simulation of retrograde gravitational capture of a satellite by Venus: Implications for the thermal history of the planet: Abstracts Volume, 26th Lunar and Planetary Science Conference, Lunar and Planetary Science Institute (Houston), p. 829-830.
Two publications on a capture origin for the Neptune-Triton system:
- Malcuit, R. J., Mehringer, D. M., and Winters, R. R., 1991, Numerical simulation of retrograde tidal capture of a triton-like planetoid by a neptune-like planet: Abstracts Volume, 22nd Lunar and Planetary Science Conference, Lunar and Planetary Institute (Houston), p. 845-846.
- Malcuit, R. J., Mehringer, D. M., and Winters, R. R., 1992, Numerical simulation of retrograde tidal capture of a triton-like planetoid by a neptune-like planet: Two-dimensional limits of a stable capture zone: Abstracts Volume, 23rd Lunar and Planetary Science Conference, Lunar and Planetary Institute (Houston), p. 827-828.
One publication on a Jupiter Orbit – Lunar Orbit resonance model:
- Malcuit, R. J., and Winters, R. R., 2001, A jupiter orbit – lunar orbit resonance model which may relate to Noproterozoic events on Earth and Moon: Geological Society of America, Abstracts with programs (National Annual Meeting), v. 33, no. 6, p. A-143.
One publication relating to the “Cool Early Earth Model”:
- Malcuit, R. J., and Winters, R. R., 2002, The cool early earth model and the lunar capture model: Are they compatible?: Geological Society of America, Abstracts with Programs (National Annual meeting), v. 34, no. 6, p. 273.
Two publications on the capture model and the primitive Earth:
- Malcuit, R. J., and Winters, R. R., 2006, The Cool Early Earth and the Tidal Capture Model: Thermal and tectonic effects on Earth and Moon: Geological Society of America, Abstracts with Programs (National Annual Meeting), v. 38, no. 7, p. 386.
- Malcuit, R. J., and Winters, R. R., 2007, Early Archean Ophiolites and the Cool Early Earth: Can they be explained in the context of a Tidal Capture Model for the origin of the Moon?: Geological Society of America, Abstracts with Programs (National Annual Meeting), v. 39, no. 6, p. 333-334.
Other articles/authors referred to in this “soliloquy” on Planetary Science:
- Bostrom, Robert C., 2000, Tectonic Consequences of Earth’s Rotation: Oxford University Press, 266 p.
- Cadogan, Peter H., 1981, The Moon – Our Sister Planet: Cambridge University Press, 391 p.
- Evans, N. Wyn, and Tabachnik, Serge, 1999, Possible long-lived asteroid belts in the inner Solar System: Nature, v. 399, p. 41-43.
- Goldreich, Peter, Murray, N., Longaretti, P. Y., and Banfield, D., 1989, Neptune’s Story: Science, v. 245, p. 500-504.
- Lada, Charles J., and Shu, Frank H., 1990, The Formation of Sun-like Stars: Science, v. 248, p. 564-572.
- Nelson, T. H, and Temple, P. G., 1972, Mainstream mantle convection: A geological analysis of plate motion: American Association of Petroleum Geologists Bulletin, v. 56, p. 226-246.
- Ross, Martin, and Schubert, Gerald, 1986, Tidal dissipation in a viscoelastic planet, in Proceedings of the 16th Lunar and Planetary Science Conference, part 2: Journal of Geophysical Research, v. B91, p. D447-D452.
- Scoppola B., Boccaletti, D., Bevis, M., Carminati, E., and Doglioni C., 2006, The westward drift of the lithosphere: A rotational drag?: Geological Society of America Bulletin, v. 118, p. 199-209.
- Singer, S. F., 1970, How did Venus lose its angular momentum?: Science, v. 170, p. 1196-1198.
- Shu, F. H., Ruden, S. P., Lada, C. J., and Lizano, S., 1991, Star formation and the nature of bipolar outflows: Astrophysical Research Letters, v. 370, p. L31-L34.
- Shu, Frank H., Najita, Joan R., Shang, Hsein, and Li, Zhi-Yun, 2000, X-Winds: Theory and Observations, in Manning, V., Boss, A. P., and Russell, S. S., eds. Protostars and Planets IV: University of Arizona Press, p. 789-813.
- Shu, F. H., Shang, H., Gounelle, M., Glassgold A. E., and Lee, T., 2001, The origin of chondrules and refractory inclusions in chondritic meteorites: The Astrophysical Journal, v. 548, p. 1029-1050.
- Taylor, S. Ross, 2001, Solar System Evolution: A New Perspective, 2nd ed.: Cambridge University Press, 460 p.
- Valley, J. W., Peck, W. H., King, E. M., and Wilde, S. A., 2002, A Cool Early Earth: Geology, v. 30, p. 351-354.
- Valley, John W., 2005, A Cool Early Earth?: Scientific American, v. 293, p. 58-65.
- Valley, John W., 2006, Early Earth: Elements, v. 2, p. 201-204.
- Ward, Peter D., and Brownlee, Donald, 2000, Rare Earth: Why complex life is uncommon in the Universe: Copernicus (Springer-Verlag, New York), 333 p.
Two recent books by the author of this note:
- Malcuit, Robert J., 2015, The Twin Sister Planet, Venus and Earth: Why are they so different?: Springer International Publishers (Switzerland), 401 p.
This book consists of 10 chapters and has abundant illustrations. Much information on the book can be accessed by googling “malcuit, twin sister planets, springer”.
- Malcuit, Robert J., 2021, Geoforming Mars: How could Nature have made Mars more like Earth?: Springer Nature (Switzerland), 420 p.
This book also consists of 10 chapters and has abundant illustrations. Much information on this book can also be accessed by googling “malcuit, geoforming mars, springer”.