Nothing comes easy to a scientist championing an unpopular idea. So Bob Malcuit wasn’t expecting popular acclaim as he stood in a conference room in Monterey, California on a December day in 1998. The conference was on the origin of the earth and moon, and Malcuit, a geology professor at Denison, was defending a definitely unpopular idea: the theory that the planetary body known as “Luna” formed somewhere in our solar system and passed close enough to be captured by Earth’s gravity some 3.9 billion years ago, becoming our moon, and in turn making it possible for life, as we know it, to flourish on Earth.
By this time, the majority of scientists believed an opposing theory, known as the giant impact model, which contends that the moon was formed as a result of collision between the earth and another planetoid. Nonetheless, Malcuit went to Monterey hoping to change a few minds. There he met with a colleague he knew fairly well, one who seemingly had an open mind about the capture theory. At this conference, though, the colleague had to level with Malcuit. He said, essentially, that the giant impact theory had the scientific stage at this point and Malcuit didn’t have much of a chance unless some new evidence emerged.
Bob Malcuit’s unrelenting adherence to the capture theory is supported by the contributions of several other scientists and some of his own students. But it’s fueled by the thousands of computer simulations of how Luna may have been captured by, escaped from, or crashed into Earth 3.9 billion years ago. It took until 2006 for Malcuit to determine what he calls the most likely scenario for how the wayfaring planetoid became our moon.
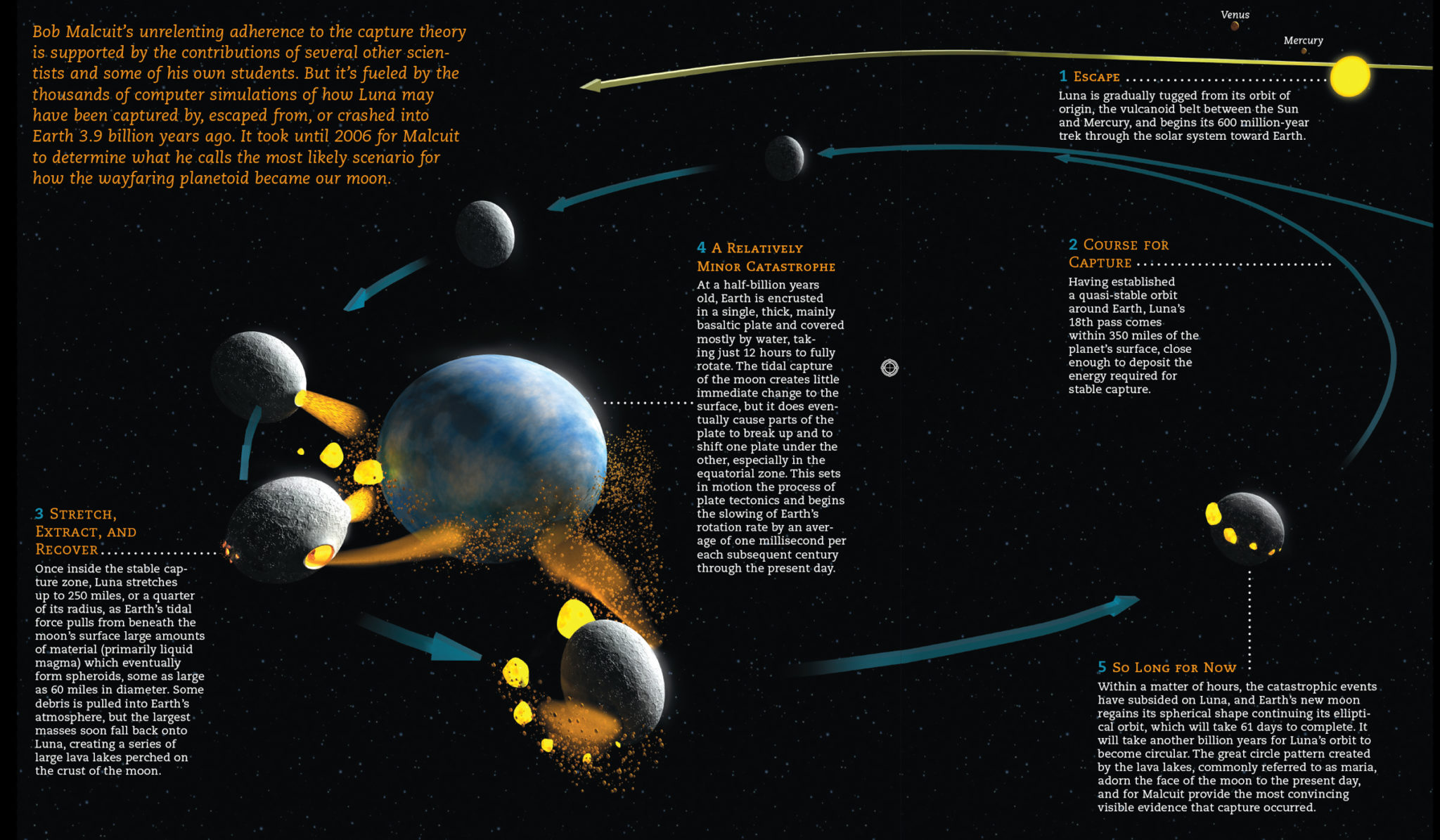
“That was pretty much the low point for me,” Malcuit says. “I was retiring the next year and it seemed like I had hit a brick wall with research on the capture model. Unless something else came along, it didn’t seem worth pursuing more work in this area.” Despite several unanswered questions surrounding giant impact, it had become the “bandwagon model,” Malcuit says. And planetary scientists were climbing onto the wagon.
It hadn’t always been this way. Before 1984, there was still quite a bit of controversy concerning where Luna came from. Several theories were orbiting about, but they all had major problems, so no one idea dominated. In fact, some scientists joked that since none of the models were completely successful, it must mean that the moon doesn’t really exist.
The giant impact model asserts that a Mars-size planetary body slammed into Earth a little more than 4.5 billion years ago, when Earth was a spry 30 million-year-old. The energy from the collision completely melted Earth’s outer layer and the impact debris went into orbit around Earth and started clumping together, eventually forming the moon. Malcuit readily points out that the giant impact theory offers only generalities and contains no specific answers for the detailed features of the moon, especially the great circle pattern of maria (märee-ah), which is the dominant feature of the face of the moon. More importantly, it doesn’t match either Earth’s or Luna’s geologic record.
It’s Malcuit’s fellow geologists—like Preston Cloud, who wrote a book in 1978 that suggested capture as the most likely model for the formation of the earth-moon system—who have taken the most collective disciplinary stand in favor of capture. They cite several pieces of evidence, like the fact that Luna’s geologic history indicates two phases of magnetization; one dates to the planetoid’s origin and the other roughly around the time of the supposed capture. They also note that the moon has no evidence of a core, thereby confounding the giant impact model, which demands a metallic core. Meanwhile, back on Earth, zircon crystals found in Australia indicate that there was ocean water on the surface as early as 4.3 billion years ago. This means that the earth’s surface was too cool for a giant impact to have occurred at the time its proponents suggest. But for all this evidence, scientists still couldn’t explain how the energy required for a capture scenario was dissipated between the earth and the moon.
An interesting footnote: geologists have not been completely alone in their stand. Two Nobel laureates—chemist Harold Urey and physicist Hannes Alfvén—and several others also asserted the capture theory in their respective books in the mid-20th century.
Nonetheless, it was in 1984, at a scientific meeting in Kona, Hawaii, that the capture theory went from one of the top contending theories to an also-ran against the giant impact model. In his book The Once and Future Moon, physicist Paul Spudis says that during the Kona conference the giant impact model “exploded into the consciousness of the community” and convinced scientists “in one fell swoop.”
Malcuit was at that conference and remembers it well. “The giant impact people had these spectacular computer simulations about the impact. They were very impressive and they convinced a lot of people. Ever since then, giant impact has been the default model.” David Kring, a visiting scientist for the Lunar Exploration Initiative in Houston, says he wasn’t even aware that there were still scientists like Malcuit who were active proponents of the capture theory. Nearly all planetary scientists accept the giant impact theory, despite its unresolved issues. “I want to say it is the consensus model, but that is almost too strong. It is certainly the preferred theory, but there are enough loose ends with it that it is still not considered definitive,” Kring says. And it is on those loose ends which Malcuit hangs his hope that one day the scientific community will accept the capture theory’s validity. If he can do that, it will be the culmination of a long journey that began in 1971 when he was a doctoral student in geology at Michigan State University. Malcuit says it all began when he purchased a lunar globe at a K-Mart in East Lansing, Michigan, on Aug. 21, 1971—“the day of discovery,” as he calls it. He was looking over his new purchase when he noticed a peculiar pattern of the maria—the large dark splotches on the moon. He noted that they formed a circular pattern around the moon, and wondered if anyone had ever noticed and written about that pattern before. While at least one other article mentioned the maria, there had been no discussion of the great-circle pattern that incorporates the main features of its face, including what we call the “man in the moon.” It was generally assumed that the spots were formed by meteor impacts. So he did some investigating, and wrote a paper about how those maria might be the result of tidal disruptions on the moon during a close encounter with the earth.
While most people are familiar with how the moon’s gravity causes tides in the earth’s oceans, tides can also occur on a planetary body’s crust. That’s exactly what Malcuit believed happened on the moon as a result of the pull of gravity from the earth. The strong pull from Earth extracted blobs of magma from beneath Luna’s surface. The blobs—some as large as 60 miles in diameter—and other debris fell back onto the surface of the moon within minutes to hours of the close encounter with the earth, resulting in a circular pattern of large lava lakes (the maria). Likewise, the gravitational force of Luna’s near pass was enough to crack the thick basaltic crust on Earth, which was mostly covered in water at that stage, and set in motion the course of events that would eventually create continents and the circumstances for living organisms to evolve.
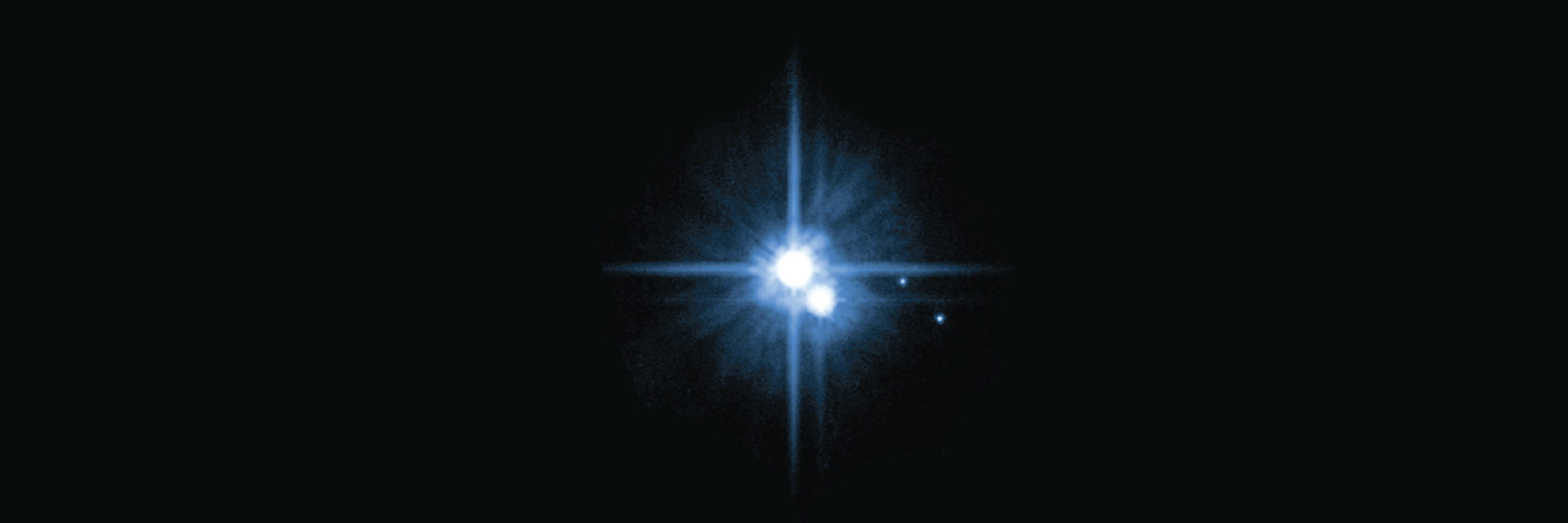
Malcuit wrote his dissertation on the topic and continued the work when he arrived at Denison in 1972. It was then that he began working with Ron Winters and Mike Mickelson, professors of physics at Denison who were intrigued by Malcuit’s questions and eager to learn more.
Malcuit abruptly ran into opposition at a spring 1972 meeting in Washington, D.C., when he presented his work for the first time. His theory was a serious challenge to the established belief that the lunar basins were formed by meteor hits, and that molten material just happened to ooze up through the lunar crust to form the maria. “For some reason, a few people in the audience did not like the idea of having lunar material…falling back on the moon to form large circular maria,” Malcuit says. “The idea seems very good to me!”
From there, Malcuit, Winters, and Mickelson began to consider the implications of the lunar capture model. Piece by piece, they began to put together the puzzle. In 1975, they presented research that showed how the capture of the moon could generate a lunar magnetic field. It was vitally important information because no one really knew how lunar rocks had become magnetized.
In 1976, they began using computer simulations of what might occur in the first encounter between Earth and Luna. “We generated a number of beautiful orbital scenarios, but none resulted in capture,” Malcuit says. “We could get the moon to circle the earth, but it always escaped. It takes a lot of energy to capture the moon and I couldn’t find a way to make it work.”
Then, in 1986, two UCLA planetary scientists showed how a planetoid the size of the moon could dissipate nearly all of the energy of tidal distortion, such as the pull on the moon by the earth. The key is that the moon had to have a certain level of viscosity—in other words it had to be stretchable. This paved the way for one of Winters’ students, David Mehringer ’88, to write a new computer code that could deal with the issue of energy dissipation, showing how the orbital energy of the moon could be converted into heat energy. Once Malcuit and his colleagues determined the conditions were favorable, they knew that capture was possible. In late September 1987, with Mehringer’s new code, the Denison researchers created the first-ever successful capture simulations. “The results were very exciting in that no one had ever demonstrated tidal capture this way before,” Malcuit says. They knew they had entered a new phase in their lunar research project, and Malcuit presented this new work at meetings from 1988 to 1990, scientists, particularly geologists, took notice.
During the following years, with the help of students like Wentao Chen ’93 and Albert Liau ’94, Malcuit produced a large number of computer simulations sometimes showing Luna passing by Earth and escaping, sometimes colliding with it, and in some instances being captured to become our moon. A pattern started emerging, leading Malcuit to define a “stable capture zone”—an area around the earth in which capture can occur if sufficient energy is dissipated between the planetary bodies. He found that there are four different stable capture zones for the earth-moon combination, and for any other planet-planetoid combination.
Malcuit admits that selling this capture scenario is not easy. “We’re talking about a catastrophic sequence of events here—but nothing like the giant impact model. That would be a thousand times more catastrophic than the highest-energy capture scenario that you can think of. And yet, giant impact is still the popular model of the present time.” Malcuit pauses a second and laughs. “You might say ours is a minor catastrophe.” The moon would bear the brunt of this “minor” catastrophe, stretching up to 250 miles under the gravitational force of the earth—about a fourth of its radius. The heat energy would melt the surface of the moon. By comparison, very little happened to the earth during the encounter. Gravity pulled rocks in the earth’s equatorial zone toward the surface about 11 miles, which is small in reference to the size of the earth.
catastrophe, and theirs is a major But that was enough to create tidal vorticity induction, or the early stages of plate tectonics and continental drift.
While the concept of stable capture zones made the capture model more realistic, it didn’t come until long after the 1984 Kona meeting where the giant impact model was essentially declared the winner. Malcuit says he has had a hard time getting anyone to pay attention since then. And by the 1998 meeting in Monterey, he was really ready to give up. Besides, his capture model still had one major problem: he didn’t have a good idea about where the moon came from. For a capture model, the place of origin of the moon was key, because it had to explain the similarities and differences between the composition of Earth and Luna.
So by 1999, when Malcuit retired from teaching, he thought he might be done with trying to convince scientists to take capture theory seriously. “I was retiring, losing my office in Olin Hall, and all my papers were being put in the attic,” he says. “I was ready to give it up and let someone else rethink the capture model.” But Malcuit never stopped reading scientific papers that related to his research. And in the past several years, studies by other researchers refueled his interest in the capture model. None of them directly address the problem of how the earth-moon system was formed, but they provide data that Malcuit was able to use to suggest the moon was formed in the inner part of the solar system, between Mercury and the Sun. One series of papers by an astrophysicist at the University of California, Berkeley, explored what is called the X-Wind Model, which discusses the very early history of the Sun and by 2003 explained the formation and composition of certain high-temperature components of meteorites, particles that should be formed in the region between the Sun and Mercury. The resulting chemical composition of these particles closely matches the moon’s composition. Another article reported work by two University of Chicago geochemists, who determined the potassium content of a number of bodies in the solar system, as well as various groups of meteorites. Few scientists referred to this research, however, because the results were difficult to explain. But in a book published in 2001, an Australian scientist argued that the study indeed provided important information, even though the giant impact model could not explain the results. In short, the potassium content of Luna suggests very strongly that it was formed between the Sun and Mercury.
Malcuit contends that the findings from these studies form the basis of a strong, perhaps convincing argument in favor of the capture theory. And, of course, for Malcuit there remained one very convincing and very visible piece of evidence. According to his calculations of stable capture zones, the moon did not have to come as close as it did for capture to occur. But the great-circle pattern of maria—seemingly too systemic for any random occurrence—serves as proof of a near miss between Luna and Earth and their subsequent, ongoing, and life-giving relationship.
So in 2004 he decided to once again join the fray. He asked the university for an office, and got his current space in the Sigma Chi fraternity house, where a number of other emeriti continue their scholarship. Now, he has a desk and computer, and is surrounded by stacks of papers, representing the thousands of capture simulations he has run. The next step, he says, is to begin trying to publish more scientific papers on the evidence he has accumulated.
But Malcuit will have to make a giant impact of his own, if he is ever going to get scientists to reconsider the capture model. His colleagues at Denison say to not count Malcuit out. “Capture is certainly not the current favored model,” Winters says. “But I think it deserves attention and eventually will get more attention.
“It’s never easy to say why a given model in science rules at a given time. But some of it has to do with the personalities of the scientists and the funding that is available. Some very powerful interests in the ’70s and ’80s favored giant impact, and to some extent that has influenced the direction the work has taken,” Winters says. “We like to think that science is not that way, but the truth is that it is. Science is no different than any other creative endeavor.”
Mickelson adds, “Science is an evolutionary process. You come up with a hypothesis and you take it where it leads you. If at some point the hypothesis doesn’t make sense, you start over and think about it again.”
Malcuit says he believes the capture model will only get back on the center of the scientific stage when the holes in the giant impact model get too big to ignore. But for now, he says, “it’s really a David and Goliath thing, and the giant impact model is the Goliath. I don’t think people will get all stirred up about the capture model until nothing else works. It has to kind of crumble, and capture to be the only good theory left, really a default model.”
It wouldn’t be the first time a theoretical Goliath was felled. Malcuit likes to compare capture theory to the theory of continental drift, which was ridiculed when it was first proposed in the early 20th century and for many decades after that. “Utter, damned rot,” was what the president of the prestigious American Philosophical Society said of continental drift when that theory first came out. But the idea later led to the concept of plate tectonics, which revolutionized Earth sciences—but not until the late 1960s. “The textbook in my beginning geology course in 1964 had one paragraph about continental drift and plate tectonics,” Malcuit remembers. “Now entire textbooks are wrapped around this idea.” People who supported continental drift and plate tectonics, he adds, “had to prove every little point to get people to believe them—every little point.” Malcuit still has his own points to prove, but judging from the encouraging findings of other scientists and his own rekindled spirit, he’s going to work like hell to do so, as sure as the moon will rise.